Q4 2020 Research Theme: A Deep Dive on Methane Emission Surveillance
- PillarFour Capital Partners
- Dec 31, 2020
- 14 min read
Updated: Nov 16, 2022
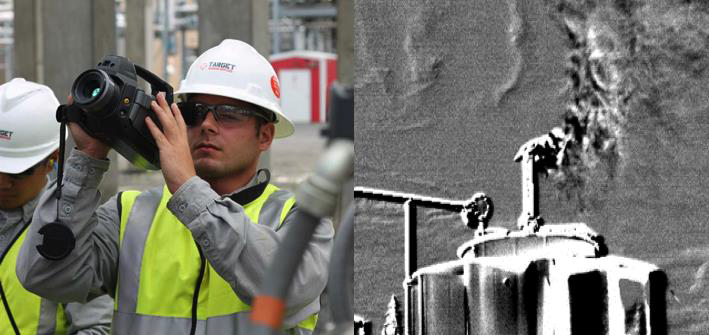
Innovation In Methane Emission Surveillance
Consistent with PillarFour’s investment strategy – scalable, proprietary, sustainable energy technologies – and the broader ‘oliving’ of oil & gas operations mentioned in this quarter’s Portfolio Briefing, we take a look at fugitive methane emissions within the oil & gas industry, emission regulations, innovative methane surveillance technologies and the increased need for emission quantification.
Methane – A Potent Yet Short-Lived Greenhouse Gas
Methane is a potent greenhouse gas (GHG), every bit as relevant to climate change as carbon dioxide. However, until recently, methane tended to receive less attention than carbon dioxide.
Existing regulations that govern industrial methane emissions typically require infrequent plant inspections(annual or semi-annual) focused on a leak detection and repair (LDAR) protocol that requires no quantification of fugitive or vented emissions, essentially a prescriptive, binary ‘go/no go’ inspection regime.
That is now changing: innovative sensor technologies, diverse surveillance platforms and powerful algorithmic processing now enable frequent, even real-time, methane
surveillance and better detection and quantification of methane emissions at lower cost.
These new methane surveillance techniques coupled with greater stakeholder awareness of methane’s potency as a greenhouse gas – long-term and short-term – are driving change within both industry and regulation.
Tighter emission regulation and ESG reporting by companies will inevitably demand quantification of methane emissions – to verify progress toward GHG targets and provide evidence for financial incentives such as tradeable GHG tax credits where applicable.
In particular, the looming 2050 deadline for a host of netzero GHG emission targets issued by governments and companies alike is focusing attention on quantitative emissions monitoring.
Regulators are likely to approve new methods and modes of quantitative methane emission monitoring – potentially triggering an inflection point in the adoption of such emissions monitoring technologies across a variety of industrial sectors – oil & gas, waste & landfill management, biogas generation and utilities.
Methane – 2nd Largest Contributor To Global Warming
The global warming potential (GWP) of methane gas is 120 times that of an equal mass of carbon dioxide. i.e. 1 kg of methane can absorb 120 times more heat energy than 1 kg of carbon dioxide when released into the atmosphere.
However, methane does not remain within the atmosphere as long as carbon dioxide, which persists for centuries; tropospheric oxidation acts as the principal atmospheric ‘sink’, converting methane to carbon dioxide – the result being that methane has an effective atmospheric half-life of some 9 years.
Despite this relatively short residence time in the atmosphere, the overall level of atmospheric methane coupled with its high initial GWP ensures that methane emissions are judged to be the second largest contributor to long-term global warming.
Global GHG Emissions By Gas, 2018
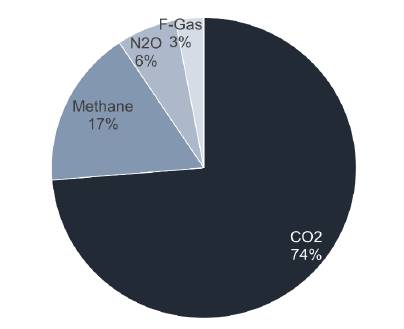
Biogenic (naturally occurring) and anthropogenic (humaninduced) sources account for ca. 40% and 60% of overall methane emissions, respectively. Of those methane emissions that are human-induced in origin, the largest contributor is agriculture – livestock and rice cultivation, closely followed by oil & gas and waste/landfill.
Human-Induced Global Methane Emissions by Source, 2019
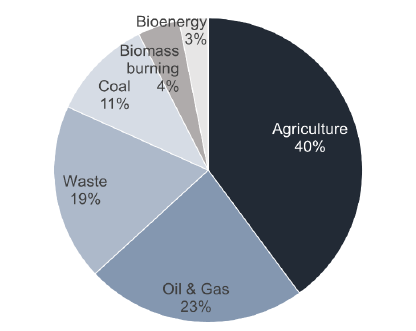
Methane GWP Varies Inversely With Timescale
Given its 9-year atmospheric half-life, methane’s global warming potential relative to that of carbon dioxide (i.e. methane’s GWP) declines rapidly over time. Over 100 years, methane is variously estimated by scientific sources to be, on average, 28-36 times as potent as that of carbon dioxide, mass for mass i.e. methane’s estimated GWP100 is ca. 28 – 36.
But, over a shorter 20-year timescale, methane is, on average, 84-87 times as potent a greenhouse gas as carbon dioxide, mass for mass i.e. methane’s GWP20 is ca. 84 – 87.
Methane’s substantially higher average GWP over shorter timescales matters and yet is often overlooked in GHG emission data published by international organizations, governments, industry bodies, companies and mainstream media commentary.
Greenhouse gas emissions are routinely ‘normalised’ and expressed in CO2-equivalent terms to enable comparisons of their respective share of overall GHG emissions.
The IEA, EU, UN, EPA, API and energy companies worldwide all apply GWP ‘multipliers’ of 25 or 30 – essentially methane’s 100-year average GWP (GWP100) in round numbers – when computing methane’s contribution to overall GHG emissions.
GWP100 Understates Benefits Of Near-Term Mitigation
With a growing intergovernmental and industrial consensus to achieve net-zero carbon/GHG targets by 2050, the need for material near-term methane mitigation grows ever stronger.
Methane’s high near-term GWP provides a fast-acting lever for GHG emission reduction and should attract much more attention.
With just 25% of any atmospheric methane emission persisting beyond 17-18 years (two half-lives), it seems perverse, indeed misleading, for regulators and industry alike to apply a GWP100 of 30 instead of the more relevant GWP20 of 85 when evaluating the GHG impact of methane emissions over the next 20 – 30 years and the potential ‘return’ on investment in surveillance technologies and equipment that can materially reduce such emissions by 2050.
GWP20 Would Better Direct Methane Mitigation Capital
In summary, the traditional GWP100 approach for restating methane emissions in CO2-equivalent terms understates the near-term impact of methane emissions on global warming and thus, in tandem, understates the potential return on investment in technology to detect, quantify and mitigate methane emissions.
Using methane’s typically quoted GWP of 30 (GWP100) and a carbon/GHG price of just US$20 per tonne CO2- equivalent (well below both the existing US$50/tonne 45Q US tax credit for CO2 sequestration and the current value of EU CO2 allowances), cost-neutrality on this GWP100 basis would require overall investments to monitor, locate and stem such gas losses be limited to less than US$11 per mcf (of fugitive/vented methane emissions).
However, a 20-year timeline and thus methane’s GWP20 of ca. 85 are frankly more relevant metrics when evaluating future near-term investments in fugitive methane abatement; using the same carbon/GHG price of US$20 per tonne CO2-equivalent, it would be deemed cost-neutral on a GWP20 basis to spend up to US$30 per mcf (of fugitive/vented methane emissions) to monitor, locate and stem such gas losses – three-fold the level of investment were methane’s GWP100 used to ‘gate’ such investments.
Both examples exclude the additional gas sale price benefit of ‘recaptured’ gas volumes once methane emissions are mitigated.
These comparative analyses do assume that existing credit and tax incentives for CO2 abatement, where applicable, will be expanded to incentivise the abatement
of methane emissions.
Furthermore, even if methane abatement is recognised within future ‘carbon’ incentive schemes, there is no certainty that governments and regulators alike will recognise and apply methane’s GMP20 when assessing methane credit and tax incentives, as opposed to
methane’s typical GWP100 reference.
Given the plethora of 2050 net-zero GHG targets, financial incentives for investment in methane mitigation should be introduced where absent and increasingly reflect the more
relevant GWP20 for methane for this shorter investment horizon.
Methane Emissions Within The Oil & Gas Industry
Whilst it is considered vital to reduce all human-induced methane emissions, there is good reason to focus on the oil & gas industry:
1) The oil & gas industry is responsible for almost a quarter of all anthropogenic methane emissions (see prior chart);
2) Methane emissions account for an estimated 57% of the oil & gas industry’s overall GHG emissions, on the basis of methane’s average overall contribution to global warming relative to carbon dioxide i.e. using methane’s GWP100 of 30.
Oil & Gas Sector – GHG Emissions By Source, % Share
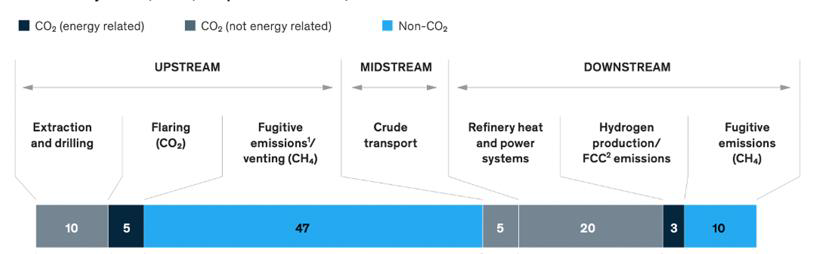
As discussed earlier, methane’s GHG potency over 20 years post-emission is almost triple its average lifetime potency.
On a 20-year timescale post-emission, methane emissions (presented on a GWP20 CO2-equivalent basis) would double overall GHG emissions and represent ca. 80% of average GHG emissions over this period – offering substantial potential for reduction via ‘low hanging fruit’.
3) Methane emissions from venting stem from known discrete equipment and facilities. Even fugitive methane emissions, e.g. leaks or incomplete flare combustion, also largely originate from known equipment: pumps, flares, valves etc.
Surveillance, detection and repair are thus relatively simple, albeit time-consuming with current field equipment, to perform for all but the most complex oil & gas facilities, including those offshore.
Upstream/Midstream Methane Emissions by Source
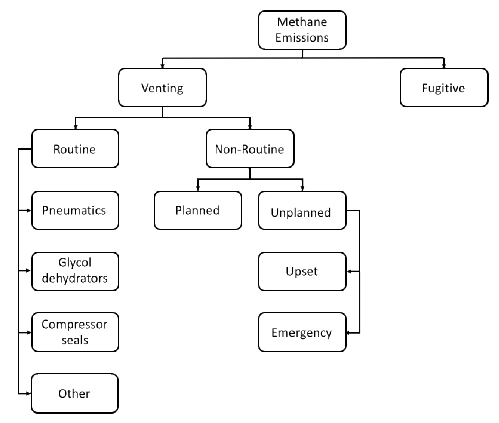
4) New methane surveillance techniques offer frequent, even real-time, cost-effective solutions for complex, remote and offshore oil & gas facilities.
5) Once captured, methane, unlike CO2, has a direct commercial value that can be readily monetised within an oil & gas facility at lower cost than within other sectors, thus helping to offset the costs of surveillance and intervention.
The IEA estimates that technical solutions exist to mitigate about 75% of current methane emissions from global oil & gas operations. Furthermore, given the direct financial benefits of reduced methane losses (and indirect benefits where GHG credits are applicable), the IEA estimates that about 40% of current methane emissions could be eliminated at no net cost, based on regional 2019 gas pricing.
Methane Emissions From Clean ‘Renewable’ Gas
On a global basis, waste and landfill generate an estimated 19% of overall human-induced methane emissions, modestly below that of the oil & gas industry. Indeed, in California, a series of airborne surveys over 2016-2018 indicate that landfill comprised the largest source of methane emissions (41%), well ahead of livestock and oil & gas, each responsible for 26% respectively.
A fast-growing global biogas industry has developed in recent years to capture and treat waste and landfill-derived methane on an industrial basis for distribution and sale as a renewable alternative to fossil-fuel based natural gas. An estimated 132,000 small, medium and large-scale bio-digesters operate globally.
However, despite the obvious ESG virtues of capturing and selling ‘renewable’ methane otherwise lost to the atmosphere, biogas facilities – just like oil & gas facilities – suffer from fugitive methane emissions, albeit typically at lower levels, from faulty pressure vents, gas piping, membrane joints and diffusion.
Emission Regulations Historically Prescriptive …
Regulatory surveillance of industrial methane emissions remains largely prescriptive in nature e.g. EPA: semiannual monitoring of leaks at compressor stations; portable optical gas imaging (OGI) cameras and VOC gas analyzers are the only EPA-approved surveillance
technologies. Furthermore, regulated leak detection and repair (LDAR) protocols currently require no quantification of emissions, essentially a binary ‘go/no go’ inspection regime.
However, the growing swathe of GHG emission reduction targets announced by companies and governments alike, whether absolute or relative, will all require accurate quantification to provide independent verification of progress toward such targets.
Current regulations governing methane emissions have yet to catch up with innovative methane detection technologies that can provide such quantification of emissions, many of which have already been invested in and/or trialled by oil & gas majors.
… But Likely To Embrace Innovative Technologies
The regulations need to adapt to technological innovation so that they do not themselves become a barrier to methane abatement.
Change is therefore afoot – regulators are increasingly eager to review and approve new and relevant technologies that enable broader, cost-effective routes to methane abatement.
… And Quantification In Turn
U.S.: For the first time in some fifteen years, the EPA recently invited operator-sponsored submissions from companies that offer innovative technologies for methane emission detection and quantification, recognising that its largely prescriptive bottom-up approach to emission regulation may no longer be fit for purpose.
Subject to a satisfactory EPA audit and approval of supporting performance data, this process will offer U.S. operators the opportunity to use these emerging, innovative technologies. EPA methane emission regulations were relaxed late 2020 by the Trump administration despite much objection from oil & gas majors – likely due to their strategic self-interest in preserving the public perception of natural gas as a ‘clean’ energy-transition fuel.
Under the new Biden administration, the EPA has until September to publish its recommended revisions to methane emission regulations. We believe it likely that innovative surveillance technologies will be approved, alongside an increased focus on methane emissions quantification.
Canada: In 2015, Alberta’s provincial government introduced legislation to cut methane emissions from upstream oil and gas operations by 45% (relative to 2014 levels) by 2025. From the get-go, innovation was viewed as critical to meeting this goal.
AER (Alberta Energy Regulator) Directive 060, which regulates fugitive methane emission surveys, requires operators to use OGI cameras and/or VOC analyzers per U.S. EPA rules. However, the directive includes an Alt- FEMP (Alternative Fugitive Emissions Management Plan) clause that allows operators to use other technology platforms e.g. drone-mounted or truck-mounted sensors, provided that equivalence with current methods has been satisfactorily demonstrated by those operators to the AER.
Regulation should not only embrace innovative technologies but also provide incentives for operators to seek innovative solutions.
Both the US and Canada currently take a prescriptive bottom-up approach to emissions reductions, in contrast to Norway’s use of a carbon tax to curb methane emissions, and Mexico’s new law which lets operators choose how to deliver facility-wide emissions reductions from a predetermined baseline.
Europe: The EU has adopted the UN-led OGMP 2.0 (Oil & Gas Methane Partnership) framework which will require accurate quantification of fugitive methane emissions from the oil & gas sector. In October 2020, the EU published its own Methane Strategy which proposes the compulsory measurement, reporting and verification of all energy related methane emissions, as well as the satellite-based detection of super-emitters. In advance of forthcoming legislation, the EU has solicited help in defining appropriate methane detection technologies, the role for emission quantification and frequency of LDAR campaigns.
In parallel with the increased oversight of oil & gas industry GHG emissions, the EU’s Regenerative Energy Directive requires that all European biogas facilities demonstrate their overall GHG savings relative to fossil fuels, thus requiring periodic surveillance and quantification of vented or fugitive methane emissions.
Methane Surveillance & Detection Technologies
As discussed earlier, methane leakage, both vented and fugitive, represents a significant proportion of overall GHG emissions.
Cost-effective detection of methane plumes, particularly from fugitive methane emissions, has become a high priority for industry and governments alike.
There are many challenges to detecting fugitive methane emissions including, but not limited to, the potential number of emission sources over wide geographical areas, the unpredictable and intermittent nature of fugitive emissions, and the broad range of leak flow rates encountered.
Furthermore, methane, the largest component of natural gas distributed to homes and businesses, is completely colourless and odourless. While mercaptan – a pungentsmelling gas – is added to natural gas at the low-pressure distribution stage so that leaks can be easily detected by consumers, upstream and midstream detection of methane emissions is more complex.
Incumbent Technologies
Optical gas imaging (OGI) currently remains the dominant regulator-approved method (e.g. EPA Quad0a) of methane detection and thus the principal technology used by oil & gas operators to satisfy regulatory and other inhouse requirements.
Optical gas imaging uses a specialised infra-red camera, typically hand-held, to render methane leaks visible onscreen. An OGI survey requires the operator to patrol a facility and survey equipment at relatively close quarters (e.g. within 6 metres under Canada’s AER Directive 060). Environmental factors, proximity and operator technique influence successful leak detection. Performing hand-held OGI surveys of well-sites and facilities is thus somewhat laborious, requiring both training and experience.
Optical Gas Imaging: Methane Surveillance
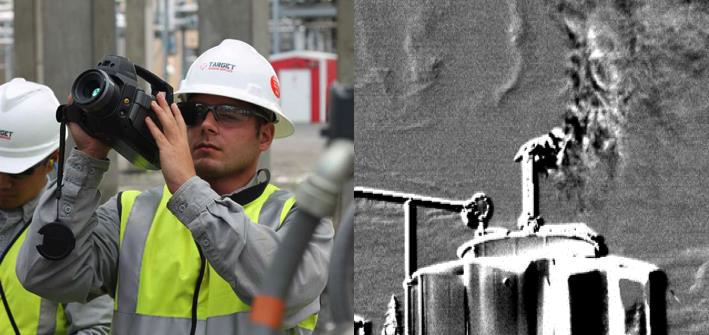
OGI technology is also not sensitive enough to detect the diffuse, low-level methane emissions observed at biogas facilities.
Furthermore, despite close proximity to any detected leak, OGI cameras are also unable to provide any quantitative measurement of a detected leak, merely detection. Quantification requires a further survey, typically using a hand-held, regulator-approved (e.g. EPA Method 21) vapour analyzer or ‘sniffer’.
Emerging Technologies
Over recent years, a variety of innovative methane detection technologies have been developed that can be variously deployed via satellite, fixed wing aircraft, drone
or ground-based.
No matter the platform, all of these rival technologies centre on methane’s ability, as with other gases, to absorb unique and specific light frequencies, typically within the infra-red spectrum, thus providing a unique, identifiable absorption ‘signature’.
These technologies can be broadly grouped into three ‘camps’, all of which boast an ability to provide quantification via algorithmic processes that require complex physics modelling.
Active Indirect: Also known as LIDAR (Laser Imaging, Detection And Ranging), these instruments project a laser beam, specifically tuned to methane’s absorption ‘signature’, across a field of interest. ‘Back-scattered’ laser energy, reflected off objects illuminated by the laser beam, is detected by a sensitive spectrometry sensor within the
instrument.
Any methane encountered by this laser beam will partially absorb its energy; the reduced level of ‘back-scatter’ laser energy is measured and the methane concentration duly quantified.
Scanning the laser beam across an area of interest can ‘map’ a fugitive emission plume and potentially identify its source.
LIDAR methane detection instruments are typically ground-based but also available on aerial platforms – light aircraft, drones.
Active Indirect: Ground-Based LIDAR Methane Surveillance
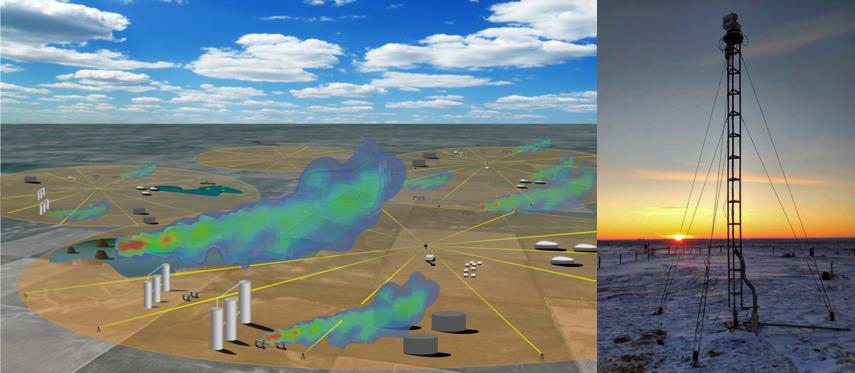
An obvious attribute of the LIDAR technique is that it is an indirect or remote measurement – no methane has to be present at the laser/sensor instrument, the laser beam need only encounter methane for detection to occur. A single areal scan – ground-based or airborne – can swiftly identify fugitive emissions for subsequent detailed follow-up and remediation.
However, such indirect ‘back-scatter’-based measurements are susceptible to the nature and
topography of illuminated objects. Known as the albedo effect, snow, water, even the choice of paint colour for structures can all influence such remote measurements – the result being false positives and negatives within a survey.
As with other remote measurements, resolution, sensitivity and an ability to provide accurate quantification are potential issues.
Passive Indirect: This technology is entirely passive in design; rather than projecting a laser beam over the survey area, natural sunlight is employed as the source of light energy. The spectrometry sensor within the instrument analyzes the reflected ‘backscatter’ of sunlight naturally incident upon objects and structures within the survey area. As with the prior indirect technique, any atmospheric methane present will ‘reveal’ itself through an anomalous reduction in ‘backscatter’ energy at precise ‘signature’ frequencies within sunlight’s broad spectrum.
With ‘backscattered’ sunlight as the source of measurement, passive spectrometry data is typically gathered from above i.e. airborne surveys. Indeed, such surveys are now provided from low-earth orbit satellites that each circle the earth in ca. 1.5 hours.
Passive Indirect: Satellite-Borne Methane Surveillance
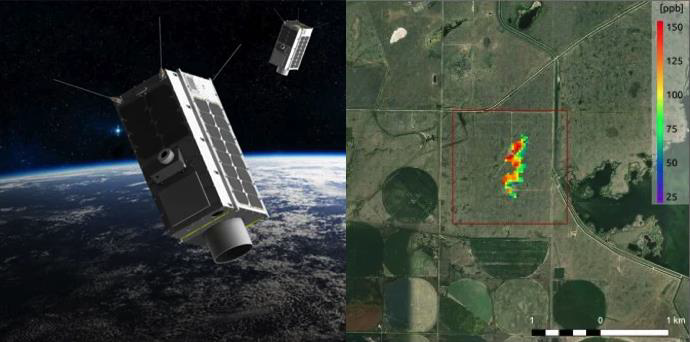
These passive indirect measurements, whether aircraft, drone or satellite-borne, inevitably share the same susceptibility as LIDAR to false positives and negatives caused by the albedo effect. Furthermore, weather conditions and the substantial offset of sensor from subject
(> 500km in the case of satellite sensors) obviously limit measurement resolution and sensitivity and thus the ability to provide meaningful location and quantification data.
However, such remote spectrometry measurements can play an important ‘triage’ role by providing cost-effective, large-scale coverage – enabling the ‘low hanging fruit’ of new or previously undetected methane ‘super-emissions’ to be rapidly identified by regulators and operators alike, pinpointed and quantified by local measurements – remote or direct – and then rectified.
Direct In-Situ: Unlike those sensor technologies that indirectly detect methane at a distance, these instruments directly measure the concentration of methane in-situ i.e. methane must enter and pass through a vented test cell. Irradiated by a tuned laser, any methane present will cause a ‘signature’ dip in the energy detected by a very sensitive spectrometry sensor, commensurate with the concentration of methane present within the cell.
This direct in-situ methane measurement takes place in a highly controlled environment, avoiding any albedo effect and ensuring a dramatic improvement in sensitivity – at parts per billion, several orders of magnitude better than indirect methane measurements – accompanied by high levels of accuracy and repeatability.
Of course, as a direct in-situ measurement, such instruments must physically traverse rather than optically scan any facility under evaluation. Therefore, such sensors are typically mounted on low-flying aircraft, drones or vehicles which then conduct periodic surveys around and downwind of facilities to enable the direct measurement and mapping of any methane emissions.
Direct, In-Situ: Drone-Borne Methane Surveillance
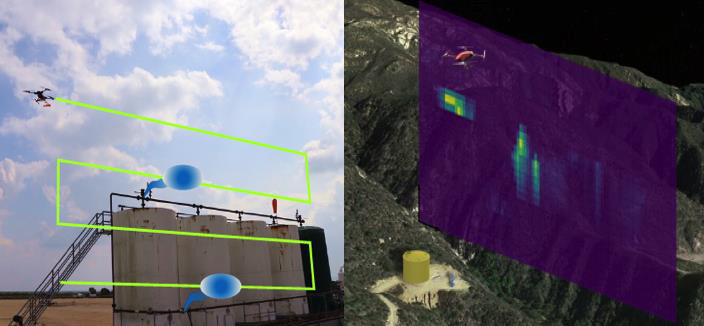
The extreme sensitivity of direct in-situ methane measurements opens up potential markets such as the surveillance of biogas facilities which, albeit subject to diffuse, lower-level methane emissions than those of the oil & gas industry, are increasingly required to validate their ESG credentials for regulators, and GHG credits where applicable.
In addition, the absence of any albedo impact on direct insitu instruments enables the accurate measurement and quantification of methane emissions from offshore oil & gas platforms, in contrast to the false ‘backscatter’ signals that often occur with remote sensing technology when over water.
Summary
The rapid evolution of sensor technologies alongside that of platforms such as microsatellites and drones are enabling swifter, better detection and quantification of methane emissions.
In a bid to drive down GHG emissions and be less prescriptive, regulators will likely approve innovative technologies that can provide cost-effective, quantitative methane emission data – triggering an inflection point in the adoption of such technology across a variety of industrial sectors – oil & gas, waste & landfill management, biogas generation and utilities.
Our industry and literature research suggests that direct insitu, 3D-spatial measurements of atmospheric methane concentration likely provide a better algorithmic base dataset for the accurate location and sensitive quantification of methane emissions.
However, no single technology or platform can or will likely address all client needs – what best suits rapid detection of a ‘super-emission’ from a remote pipeline will likely differ to that for routine quantitative regulatory data for onshore facilities or indeed a survey of an offshore platform in the Gulf of Mexico. Clients will select and combine methane detection technologies that best address their specific needs in the most cost-effective manner
Download the PDF
This material is intended for information purposes only. This material is based on current public information that we consider reliable, but we do not represent it as accurate or complete, and it should not be relied upon as such. We seek to update our research as appropriate, but various regulations may prevent us from doing so.
Estimates, opinions and recommendations expressed herein constitute judgments as of the date of this research report and are subject to change without notice. PillarFour Capital Partners Inc. does not accept any obligation to update, modify or amend its research or to otherwise notify a recipient of this research in the event that any estimates, opinions and recommendations contained herein change or subsequently become inaccurate or if this research report is subsequently withdrawn.
No part of this material or any research report may be (i) copied, photocopied or duplicated in any form by any means or (ii) redistributed without the prior written consent of PillarFour Capital Partners Inc.
Website links or e-mail communications may contain viruses or other defects, and PillarFour Capital Partners Inc. does not accept liability for any such virus or defect, nor does PillarFour Capital Partners Inc. warrant that e-mail communications are virus or defect free.
This document has been approved under section 21(1) of the FMSA 2000 by PillarFour Securities LLP (“PillarFour”) for communication only to eligible counterparties and professional clients as those terms are defined by the rules of the Financial Conduct Authority. Its contents are not directed at UK retail clients. PillarFour does not provide investment services to retail clients. PillarFour publishes this document as a marketing communication and NOT Independent Research. It has not been prepared in accordance with the regulatory rules relating to independent research, nor is it subject to the prohibition on dealing ahead of the dissemination of investment research. It does not constitute a personal recommendation and does not constitute an offer or a solicitation to buy or sell any security. PillarFour consider this note to be an acceptable minor non-monetary benefit as defined by the FCA which may be received without charge.
This note has been approved by PillarFour Securities LLP (FRN 722816) which is authorised and regulated by the Financial Services Authority.